Overview
Marine ecosystem productivity depends on the amount of primary production by phytoplankton, unicellular photosynthetic algae and cyanobacteria suspended in the water column that form the base of the marine food web. Measurements of the primary photosynthetic pigment, chlorophyll a (CHL), are commonly used as a proxy for phytoplankton biomass. Near-surface CHL can be measured remotely by ocean color sensors on satellites and then incorporated into integrated models to estimate primary production (PP). Phytoplankton composition and changes in the timing (phenology) and magnitude of blooms (peaks in biomass), can provide sensitive indicators of ecosystem responses to major external disturbances (Song et al. 2010). The Northeast Large Marine Ecosystem (NE-LME) may be particularly vulnerable to phenological shifts that are in response to climate change because recruitment success of higher trophic levels is highly reliant on synchronization with pulses and composition of phytoplankton production (Cushing et al. 1990, Edwards & Richardson 2004). Blooms of certain toxin producing phytoplankton can also result in harmful algal blooms (HABs) that can negatively impact the health of other organisms, including humans.
- Gulf of Maine
There is an onshore to offshore gradient of decreasing PP in the Gulf of Maine, with the lowest PP in the NE- LME occurring over the deep basins. Mean seasonal patterns include low concentrations during winter, except along coastal margins and shallow banks, a region-wide spring bloom followed by a mid-summer decrease and often a fall bloom (Thomas et al. 2003). Variations within the GOM are often related to patterns of vertical stability and nutrient sources.
- Georges Bank
Primary production rates on Georges Bank are among the highest of any continental shelf seas (O'Reilly et al. 1987). Relatively high phytoplankton concentrations are consistently found within the shallow (< 60 m), tidally well-mixed regions of Georges Bank with mean concentrations equivalent to those observed in nearshore areas (NEFSC 2018). Strong currents generated by diurnal and semidiurnal tides interacting with the shallow bottom topography keep the shallow portions of Georges Bank mixed throughout the year (O'Reilly & Zetlin 1998) and help support an extensive food web including high levels of fish production that have sustained commercial fisheries since the 16th century (Fogarty and Murawski 1998).
- Mid-Atlantic Bight
The Mid-Atlantic Bight is a relatively productive continental shelf system supporting a wide variety of fauna and flora, including commercially important shellfish and groundfish. Primary productivity is highest in the nearshore and estuarine regions with coastal phytoplankton blooms initiating in the fall and winter, although the timing and spatial extent of blooms varies interannually. Offshore, strong stratification during the summer limits nutrient inputs to the surface layer, often resulting in subsurface chlorophyll maxima that are not detectable by satellite remote sensors. Near the shelf-break, a thermohaline front develops during summer upwelling deep, nutrient rich waters to the surface and supporting localized enhanced phytoplankton biomass that propagates up the food web generating local patches of zooplankton, fish and top predators (Genin 2004).
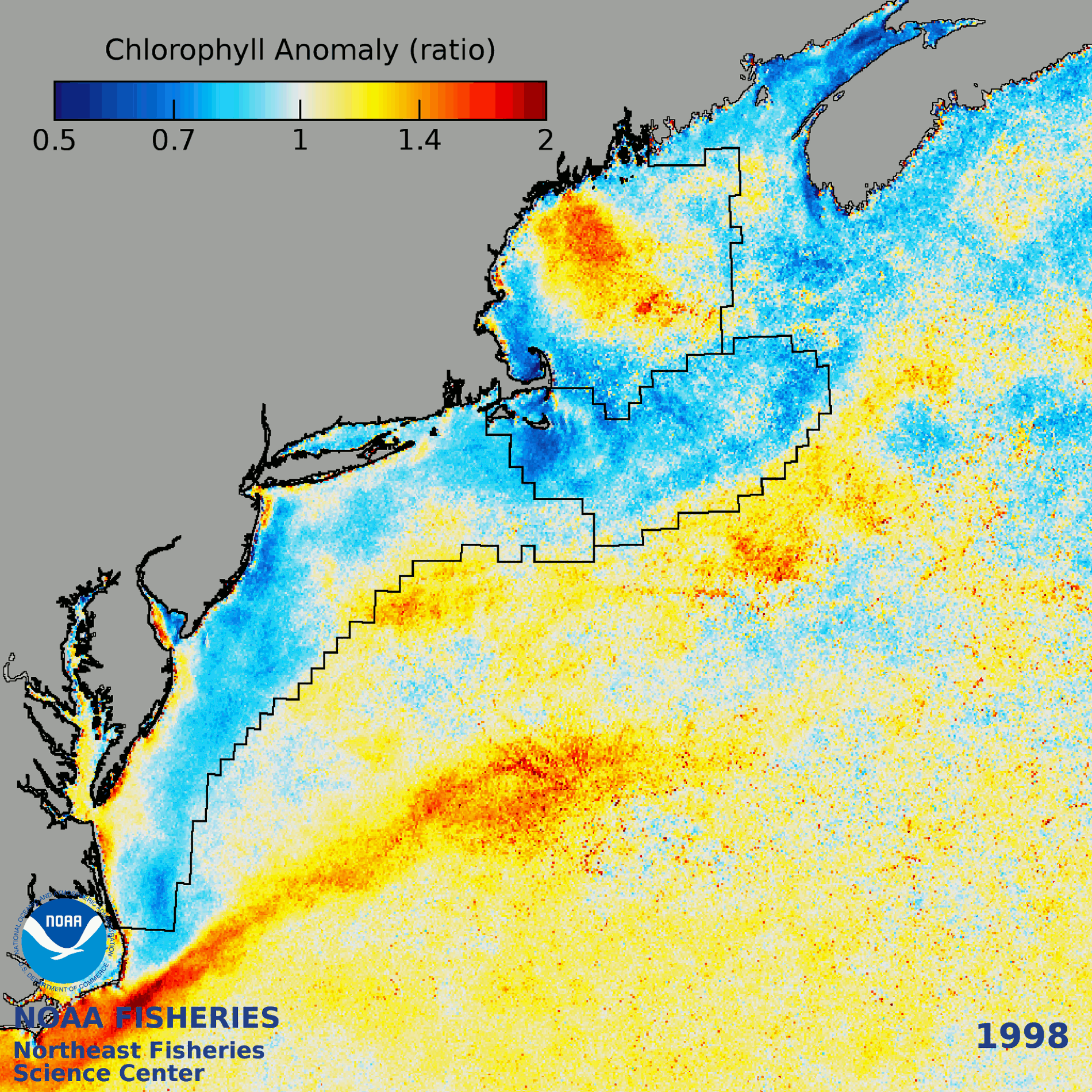
Ecological Interactions
The NES LME is a highly productive continental shelf ecosystem, which supports several important commercial fisheries (O'Reilly et al. 1987, Link et al. 2008). Winter convective mixing, vertical tidal mixing and estuarine outflows supply nutrients into surface waters supporting phytoplankton production (Townsend et al. 2006) and influencing trophic food-web dynamics. Strong correlations between primary production and marine fish production (Ryther 1969, Iverson 1990) and their fundamental role in the global carbon cycle make primary producers significant components of the marine ecosystem. Thus it is critical to consider phytoplankton in ecosystem based management plans and to understand how changes in the phytoplankton community and its productivity can alter the structure and function of marine ecosystems.
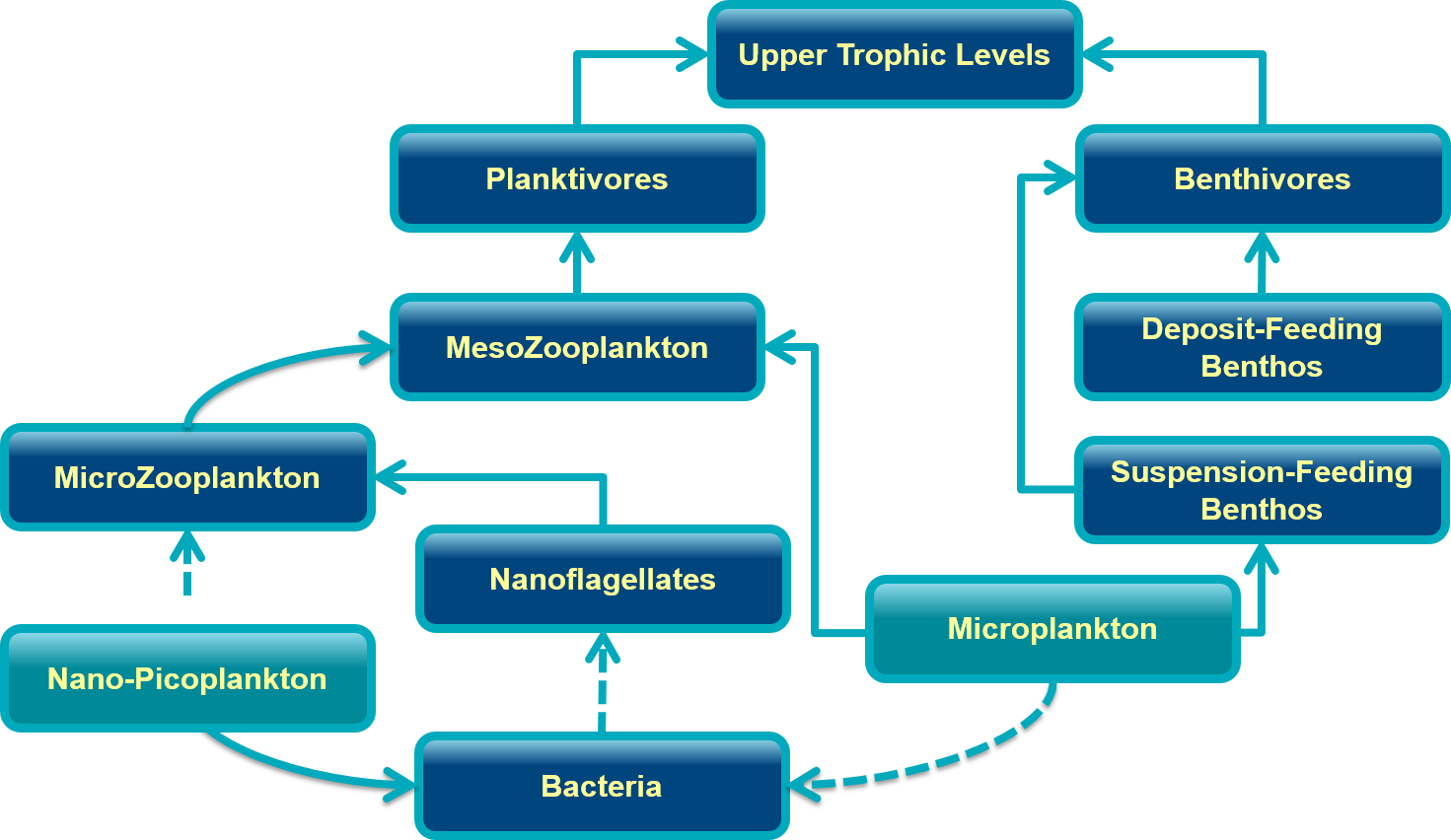
Environmental Drivers
Marine phytoplankton biomass, production and composition are driven by changes in the environmental forcings (bottom-up control) and consumers (top-down control). Changes in day length, localized weather events, regional currents, water column stratification and mixing, and basin-scale climate patterns alter the physical environment, subsequently affecting overall phytoplankton production and seasonal variability. The seasonal cycle of phytoplankton growth, composition, and biomass s strongly correlated with dissolved nutrient concentrations in the euphotic zone. During winter, nutrients accumulate in the surface waters when lower light levels limit phytoplankton growth. As growing conditions improve in spring, uptake by phytoplankton reduces nutrient concentrations and summer stratification limits mixing of deep water nutrients to the surface. During summer, productivity is at its highest, but is reliant on recycled nutrients within the water column by smaller phytoplankton species (i.e. the microbial loop). Despite high primary productivity during the summer, phytoplankton biomass is actually low; however localized upwelling events or storms can periodically mix deep water nutrients to the surface creating episodic phytoplankton blooms.
- Gulf of Maine
In the GOM, the principal supply of nutrients supporting new primary production are from the cold, fresh Labrador Sea Slope Water and the warmer and saltier Warm Slope Water originating from the Gulf Stream. The nitrate load in the Warm Slope Waters is approximately 50% higher than that of the Labrador Slope Waters, which can alter the structure and function of the planktonic ecosystem, including the cell densities of the toxic dinoflagellate Alexandrium fundyense (Townsend et al. 2010, Townsend et al. 2014).
- Georges Bank
On the shoals of GB, vigorous tidal mixing injects deep nutrient rich water onto the Bank, which is then dispersed and advected to drive phytoplankton growth throughout the year. In the shallow central portion of GB, light is not a limiting factor of photosynthesis, thus winter concentrations of phytoplankton are often higher than the surrounding areas. Following the winter-spring bloom, cross-frontal nutrient fluxes on the northwest flank to higher phytoplankton concentrations that are advected around the Bank to the southern flank, fueling secondary production and leading to higher stocks of zooplankton in that region (Townsend et al. 2006).
- Mid-Atlantic Bight
The MAB is characterized by well-mixed, light-limited, nutrient replete conditions in the winter, and stratified, nutrient-limited, conditions in the summer. Near-shore upwelling, river plumes, and shelf break processes create seasonally varying production gradients through different physical mechanisms (Mouw et al. 2005). At the shelf break, retention of upwelled offshore nutrients enhances summer phytoplankton production, aggregating and attracting lower and mid-trophic level species, and thus creating persistent and predictable foraging areas for marine predators (Thorne et al. 2017).
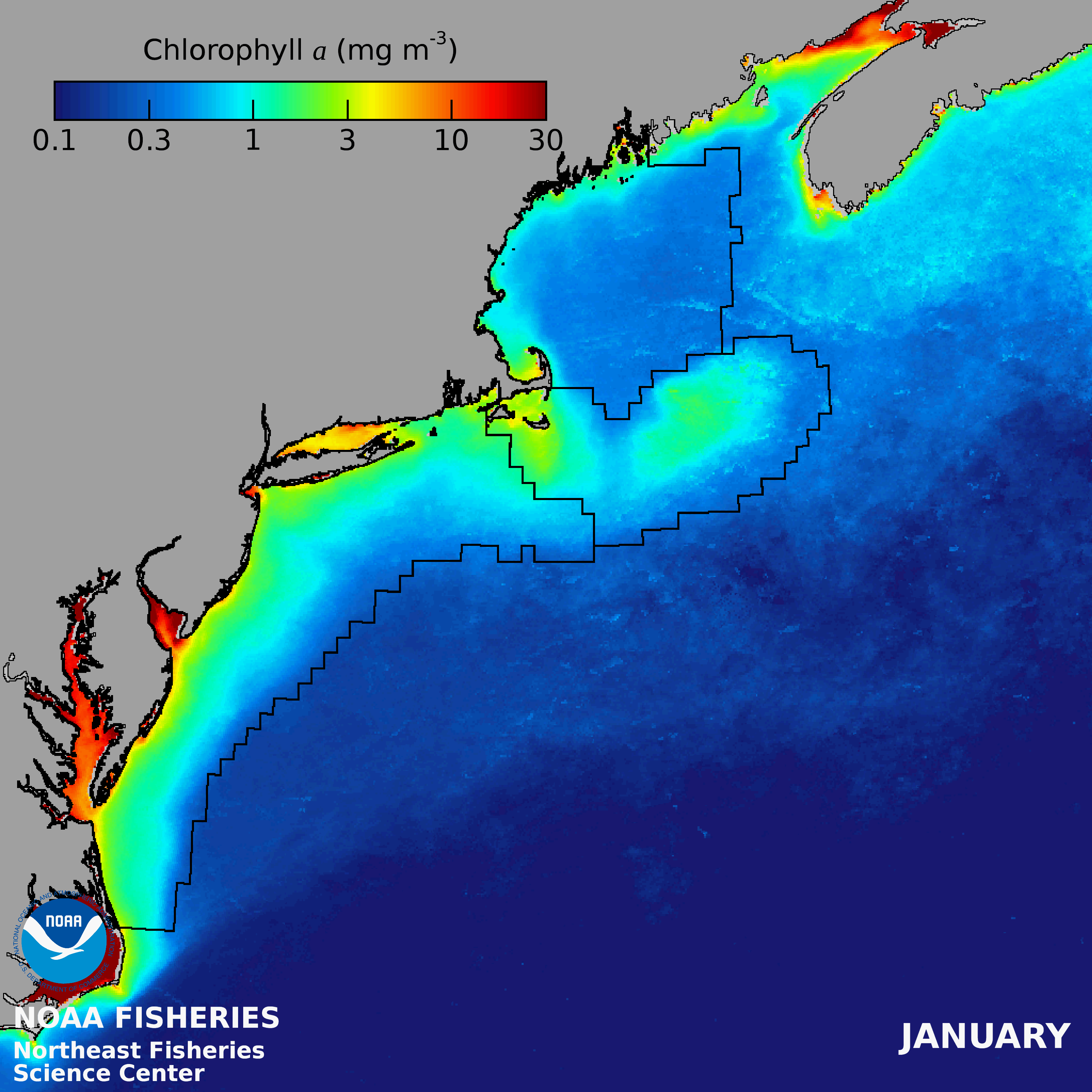
Human Activities
While high abundances of phytoplankton typically provide increased food for secondary production, some phytoplankton species can negatively impact human activities and other higher trophic levels. Collectively these occurrences are called Harmful Algal Blooms (HABs) and can cause significant impacts to fisheries and human health.
- Alexandrium spp.
Toxic dinoflagellate blooms of Alexandrium occur annually and produce saxitoxin and related neurotoxins. Filter feeding bivalves can accumulate the toxins, causing Paralytic Shellfish Poisoning (PSP) when consumed by humans. All states in the NE have rigorous monitoring programs to insure that commercially available shellfish are safe to eat. Blooms in coastal waters of the Gulf of Maine are typically found first off the coast near Portland, Maine and southward along the coast to Cape Ann in late May and early June. Cell densities are highest in eastern Maine later in summer, in July and August, and blooms in the Bay of Fundy can occur as late as September and October. Both inshore blooms and offshore blooms, which remain largely undetected, can cause mortalities of fish marine mammals, turtles and birds (Starr et al. 2017).
- Pseudo-nitzschia spp.
Domoic acid, produced by the some diatoms of the genus Pseudo-nitzschia, accumulates in filter-feeding bivalves and fish and causes amnesic shellfish poisoning (ASP) in humans and can lead to fatalities of marine mammals and seabirds (Lefebvre et al. 2002, Fernandes et al. 2014). Blooms of Pseudo-nitzchia have become more common in the past decade causing closures of shellfish beds in Maine and Rhode Island in 2016 and 2017 (NEFSC 2018).
- Other HAB species
In the lower Chesapeake Bay, annual blooms of the dinoflagellate Cochlodinium polykrikoides have been observed for several decades and more recently, blooms of Alexandrium monilatum, a toxin-producing dinoflagellate common to the Gulf of America (formerly the Gulf of Mexico), have invaded the region. Both dinoflagellate species have been associated with fish kills either directly or indirectly, and may also negatively impact shellfish aquaculture. Blooms of C. polykrikoides and Aureococcus anophagefferens (responsible for “brown tides”) occur regularly in the Long Island Sound region, with the most extensive and longest-lasting brown tide event ever recorded occurring in 2017.